This is the fourth installment in the Enzymes for Brewers series.
Biochemists envision enzyme-catalyzed reactions as occurring in two steps. First there’s the formation of the enzyme-substrate complex. Then there is the subsequent formation of the product.
In most realistic situations — and certainly in brewing — the concentration of the enzyme is small compared to that of the substrate. As the concentration of substrate rises, so does the reaction rate, as the average distance between enzyme and substrate is reduced. Put more simply, in a brewing-relevant example, the more starch there is in the mash, the more likely an amylase enzyme floating in the liquid is to bump into a strand of it. At some point, however, enzyme activity does not increase as all the enzyme is tied up in enzyme-substrate complexes.
Substrate Concentration
This relationship between substrate concentration and reaction rate is given by the Michaelis-Menten equation. Basically, Michaelis and Menten showed that increasing the concentration of a substrate initially greatly increases the rate of reaction. Beyond a certain point, however, the rate increase slows with increasing substrate concentration. The rate is still increasing, just not as fast as did at lower substrate concentrations. This relationship can be shown graphically as a curve that starts at zero, initially climbs quickly, but then slowly approaches the maximum rate of reaction (called Vmax).
Although the Michaelis-Menten equation is useful for learning some basic ideas about enzyme kinetics, applying it numerically to brewery mashes would likely be difficult (and, for practical purposes, pointless). For one thing, in the case of starch degradation, the substrate concentration is rapidly decreasing and reaches nearly zero at the end of the mash. In addition, the enzyme concentration — assumed to be constant in the Michaelis-Menten curve — is also decreasing as the enzymes (especially beta amylase) are being denatured during the mash. Furthermore, starch is mainly degraded by two enzymes, and the proportions of these change over the course of the mash. Beta amylase is initially over twice as abundant as alpha amylase, but its concentration decreases steadily over the course of a mash. Alpha amylase levels decline more slowly, especially at lower mash temperatures. So, at the end of a long mash, beta amylase may be almost completely inactivated while some alpha amylase activity is still occurring on the tiny amount of starch remaining.
Additionally, with large biological molecules, another factor can come into play. At high substrate concentrations, enzyme activity can be slowed by physical constraints. The enzyme can be trapped in “pockets” that are surrounded by parts of the substrate it can’t bind to. In brewing, this can occur in very thick mashes. Beta amylase (the enzyme) can temporarily be trapped in a “cage” of amylose strands (the substrate), unable to find a reducing end to bump into. Both amylase enzymes can also suffer from competitive inhibition in thick mashes. The amylase enzymes bind to their substrate (starch), but they will also temporarily bind to their products (the simpler sugars) as the shape of these sugars is a reasonably good fit to at least part of their active site. Finally, in very thick mashes, there might not be enough free water for the enzymes to float freely in solution.
Enzyme Concentration
Increasing the concentration of enzymes will also speed the overall formation of product. Since the enzyme concentration is low compared to the substrate, an increase in the concentration of enzymes will usually cause a linear increase in the formation of the product. For example, if you double the enzyme concentration, the reaction rate will double.
Brewing-Relevant Aspects
In the mash, the brewer controls the concentration of the substrates through various means. There are a variety of molecules from barley malt that are substrates for enzymes in the mash. These include gums, protein, and starch. The concentration of these can be influenced by malt selection. For example, the levels of proteins (which can be a substrate for proteases in a step mash) can be increased by choosing high-protein malts (such as wheat malt), or decreased by adding low-protein adjuncts — such as corn or rice – to the grist. Likewise, wheat and rye malt have more beta glucans (a type of gum) than barley malt, and these may be acted on by beat-glucanase at low temperatures rests. Obviously, the primary consideration when choosing malts is the type of beer desired. The effect on substrate and enzyme concentrations is secondary. In addition, the primary factor that determines substrate concentration is mash thickness.
Mash Thickness
The concentration of starch (and other molecules that come from malt) in the malt is primarily determined by the mash thickness.
At the beginning of a mash, when both the substrate concentration and enzyme concentration are at their greatest, rate of reaction is relatively fast. Near the end of a mash, when the substrate concentration is close to zero – and the enzyme concentration may have dropped below a quarter of its initial value — the reaction rate is greatly slowed. The Michaelis-Menton curve gives us an idea of how enzymes should react to changes is concentration ideally, but is not of any practical use for a brewer as things such as competitive inhibition and physical contraints at high substrate concentrations come into play.
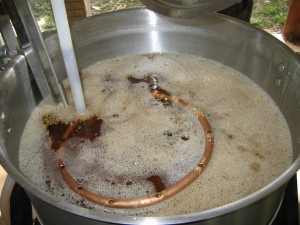
In real-world homebrewing situations, a wide range of mash thicknesses will yield acceptable results.
In homebrewing, a mash thickness of 1.25 qts. of water per pound of grain (2.6 L/kg) is very common. This relatively thick mash mirrors common English brewing conditions and is also helpful to brewers with limited mash tun space. Thicker mashes may be slower to convert and yield less extract. Mashes thicker than 1.0 qts.lb. (2.6 L/kg) are likely to yield unacceptably poor results. It’s interesting to note that a small amount of water is consumed during the mash. When starch is degraded into simple sugars, this is done via hydrolysis — the adding of a water molecule to the starch molecule to break a chemical bond. Thus, the mash needs to be thin enough initially so that water lost to absorption into grain solids and hydrolysis does not yield an exceptionally thick mash. The common homebrew thickness of 1.25 qts./lb. (2.6 L/kg) is very workable, but near the thick end of the “window” of acceptable mash thicknesses. [Mashes as thin as 2.4 qts./lb. (5.0 L/kg) are used in some commercial breweries. For breweries that pump their mash from vessel to vessel — as in the case of breweries with a separate mash and lauter tuns, or when decoction mashing — thinner mashes are preferred.]
At normal mashing temperatures, progressively thinner mashes give slightly more fermentable worts — the opposite of what the Michaelis-Menten curve would predict. Oddly enough, at high mash temperatures — higher than 167 °F (75 °C) — thinner mashes yield less fermentable worts, which falls in line with the ideas behind the Michaelis-Menton curve. The effect of mash thickness on fermentability, however, is less than the effect that temperature has. For this reason, most brewers rely on mash temperature to set their wort fermentability.
Diastatic Power
Brewers can alter the concentration of enzymes in their mash by choosing malts with different diastatic powers (DP). (Diastatic power is a measure of the activity of the starch degrading enzymes, working together. The activity of alpha-amylase alone is also sometimes given on malt analysis sheets.) For example, a two-row malt produced in the United States, with a DP of 120, should convert the starches in a mash twice as fast as an English pale malt with a DP of 60. In brewery settings, higher DP malts do indeed convert their mashes faster, as indicated by an iodine test. Enzyme concentrations in mashes are diluted when adjuncts or specialty malts are used.
So, essentially, you have a fairly wide window of mash thicknesses that will yield acceptable results. Anywhere from 1.2 qts./lb. (2.5 L/kg) to 2.4 qts./lb. (5.0 L/kg) should be fine. In thicker mashes, there will not be enough free water to float the enzymes. The rate of reaction will also be lowered via competitive inhibition and physical constraints. In mashes thinner than 2.4 qts./lb. (5.0 L/kg), the substrates and enzymes may be far enough away from each other that the rate of reaction could be greatly slowed. Although mash thickness plays a role in wort fermentability, conflicting results are seen at different temperatures. As such, brewers mainly seek to control the fermentability of their wort through choosing the proper mash temperature.
In the next article in this series, that I will post tomorrow, we will explore the effect of temperature on enzyme kinetics.
—
Related articles
The last sentence under MASH THICKNESS states, “For this reason, most brewers rely on fermentation temperature to set their wort fermentability.”
The mash temperature and rest time would determine wort fermentability, not fermenation temperature. Correct?
Whoops, it should have read “mash temperature,” not “fermentation temperature.” Braino. Fixed.